Key Publications
Interface-Induced Renormalization of Electrolyte Energy Levels in Magnesium Batteries
A promising strategy for increasing the energy density of Li-ion batteries is to substitute a multivalent (MV) metal for the commonly used lithiated carbon anode. Magnesium is a prime candidate for such a MV battery due to its high volumetric capacity, abundance, and limited tendency to form dendrites. One challenge that is slowing the implementation of Mg-based batteries, however, is the development of efficient and stable electrolytes. Computational screening for molecular species having sufficiently wide electrochemical windows is a starting point for the identification of optimal electrolytes. Nevertheless, this window can be altered via interfacial interactions with electrodes. These interactions are typically omitted in screening studies, yet they have the potential to generate large shifts to the HOMO and LUMO of the electrolyte components. The present study quantifies the stability of several common electrolyte solvents on model electrodes of relevance for Mg batteries. Many-body perturbation theory calculations based on the G0W0 method were used to predict shifts in a solvent’s electronic levels arising from interfacial interactions. In molecules exhibiting large dipole moments, our calculations indicate that these interactions reduce the HOMO–LUMO gap by ∼25% (compared to isolated molecules). We conclude that electrode interactions can narrow an electrolyte’s electrochemical window significantly, thereby accelerating redox decomposition reactions. Accounting for these interactions in screening studies presents an opportunity to refine predictions of electrolyte stability.
Surface-mediated Solvent Decomposition in Li-air Batteries: Impact of Peroxide and Superoxide Surface Terminations
A viable Li/O2 battery will require the development of stable electrolytes that do not continuously decompose during cell operation. Recent experiments suggest that reactions occurring at the interface between the liquid electrolyte and the solid lithium peroxide (Li2O2) discharge phase are a major contributor to these instabilities. To clarify the mechanisms associated with these reactions, a variety of atomistic simulation techniques, classical Monte Carlo, van der Waals-augmented density functional theory, ab initio molecular dynamics, and various solvation models, are used to study the initial decomposition of the common electrolyte solvent, dimethoxyethane (DME), on surfaces of Li2O2. Comparisons are made between the two predominant Li2O2 surface charge states by calculating decomposition pathways on peroxide-terminated (O22–) and superoxide-terminated (O21–) facets. For both terminations, DME decomposition proceeds exothermically via a two-step process comprised of hydrogen abstraction (H-abstraction) followed by nucleophilic attack. In the first step, abstracted H dissociates a surface O2 dimer, and combines with a dissociated oxygen to form a hydroxide ion (OH–). The remaining surface oxygen then attacks the DME, resulting in a DME fragment that is strongly bound to the Li2O2 surface. DME decomposition is predicted to be more exothermic on the peroxide facet; nevertheless, the rate of DME decomposition is faster on the superoxide termination. The impact of solvation (explicit vs implicit) and an applied electric field on the reaction energetics are investigated. Our calculations suggest that surface-mediated electrolyte decomposition should out-pace liquid-phase processes such as solvent auto-oxidation by dissolved O2.
Crystal Surface and State of Charge Dependencies of Electrolyte Decomposition on LiMn2O4 Cathode
First principles calculations are used to study the initial decomposition reactions of ethylene carbonate (EC) on the (111) surface of LiMn2O4 (LMO), a candidate for Li ion battery (LIB) cathode. Theoretical studies of interfacial reactions are particularly timely due to recent experiments on the effect of LMO crystal morphology on battery cyclability and interfacial film stability [J.-S. Kim, K. Kim, W. Cho, W. H. Shin, R. Kanno, and J. W. Choi, Nano Lett., 12, 6358–6365 (2012)]. We find that EC degradation is a two-step reaction. The first step is the rate determining reaction where a proton is abstracted from EC and transferred to the surface. The second step involves ring opening of the proton abstracted EC which turns out to have a smaller barrier. Both of these reactions are sensitive to the Li content, i.e. state of charge, of the model electrode. EC degradation via H-abstraction becomes a feasible reaction route when the cathode becomes more highly charged. Comparison with predictions on the (100) surface of LMO is discussed, and speculation on the growth of nanometer-thick interfacial layer on LMO despite its relatively modest operating potential are made in light of the reaction driving forces predicted in this work.
Currently, I am a postdoctoral research fellow at the University of Michigan in the Mechanical Engineering department working with Prof. Donald J. Siegel.
My interest lies in using theoretical models to understand the fundamental processes occurring in complex systems. We have been working on several battery chemistries (Li-S, Mg, Li-air, Li-ion) that have the potential to increase capacity and reduce cost.
In the course of my research, I have gained experience with vast number of simulation techniques. To name a few, DFT for electronic structure, Green's Function technique for transport properties, LAMMPS and ReaxFF for classical simulations.
About
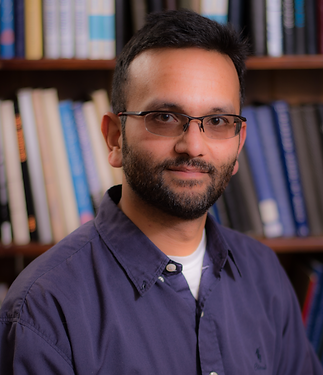
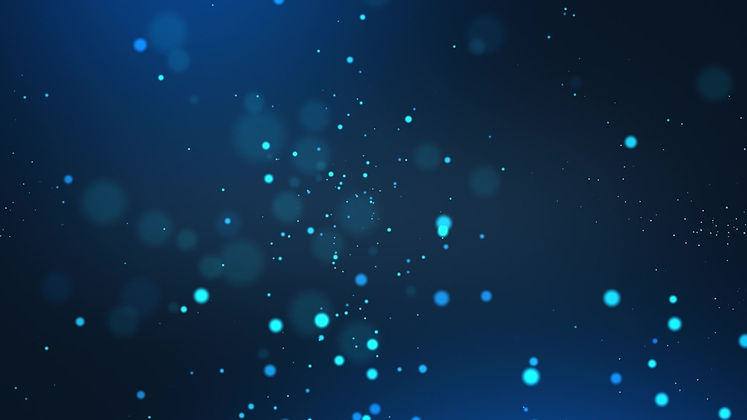